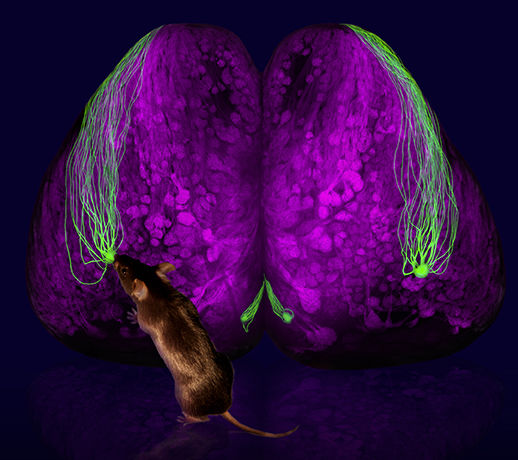
We study how olfactory information is represented and processed in neural activity patterns, and how the brain extracts information relevant for behavior.
We are using wide-field and two-photon imaging, multiunit electrophysiology and behavioral experiments, in combination with theoretical approaches, to study how odors are represented at different stages of neural processing. To understand how spatiotemporal neural codes are read by the brain and evoke behavior, we are developing optogenetic pattern stimulation techniques, including 2P holography. Most of the projects are deeply rooted in theoretical approaches and statistical modeling. In addition, as an applied direction, we are developing bio-electronic nose, a universal, versatile, and sensitive chemical detector based on the mouse olfactory system.
Current projects
Basic principles of the olfactory coding
Understanding sensory processing relies on establishing
a consistent relationship between the stimulus space, its
neural representation, and perceptual quality. Based on
the recently proposed primacy model for concentration
invariant odor coding, and a few simplifying assumptions,
we have developed a theoretical framework for mapping
the odor input space to the response properties of
olfactory receptors (Giaffar-2017). The theory predicts
specific relationships between receptor fields of
individual receptor types (or glomeruli), the statistical
features of mitral/tufted cell feedforward connectivity
to the cortex, processing of odor mixtures, and
structure of perceptual odor space.
Currently we are developing both experimental and computational approaches to test multiple assumptions and predictions of the theory. We are interrogating the olfactory system at different levels. This includes, but not limited to, wide-field imaging with high temporal resolution of glomeruli odor maps, two-photon imaging and multi-unit electrophysiology of mitral/tufted cells, multi-unit recordings in piriform cortex, optogenetic pattern stimulation, and qualitative behaviors.
We are part of the BRAIN Initiative funded multi-lab consortium: “Cracking the Olfactory Code”
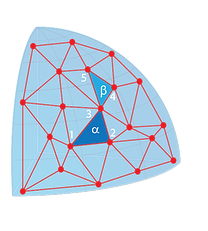
Representation of the odor identities alpha and beta by activation of the sets of primary (the most sensitive) receptors {1,2,3} and {3,4,5} as simplexes on the low dimensional manifold in the space of the receptor affinities to different ligands.
Behavioral relevance of neuronal code features
The most powerful way to test a neural coding model is to validate that the hypothesized code can be read by higher brain areas where it leads to an observable behavioral output. To this end, we are developing experimental strategies to establish causal links between the neuronal code and animal behavior. Following our earlier work with single glomerulus stimulation (Smear-2013) we are developing a general approach to test the readability of the olfactory code using an optogenetic pattern stimulation system in behaving mice. In this paradigm, mice are trained to recognize a specific spatial-temporal pattern of glomerulus activation. Through systematic perturbation of this pattern during measurements of the behavioral response to these perturbations, we investigate the role of different features of the code for animal behavior.
The next step after dissecting the olfactory code at the glomerulus/receptor level is testing of neural coding models at the individual neuron level. This requires developing a different technology capable of individual neuron stimulation. In collaborative project with the Shoham lab (Tech4Health, NYU) we are developing a system for 2-photon holographic optogenetic stimulation of multiple neurons deep in the brain of the behaving animal.
To generalize our approaches to other sensory modalities we participating in the BRAIN Initiative funded consortium: “Readout and control of spatiotemporal neuronal codes for behavior”
Bio-electronic nose
We are developing a universal, versatile, and sensitive chemical detector based on the mouse olfactory system. For years, trained animals were used for chemical detection in homeland security, defense, healthcare, agriculture, and other applications, because a biological olfactory system outperform all artificial chemical detection devices, especially when it comes to simultaneous versatility, speed, and specificity in detecting volatile chemicals. However, the use of animals in this capacity requires extensive training and behavior-based communication. Here we are developing an alternative strategy, that bypasses behavioral output by reading olfactory information directly from the brain. We use brain-machine interface that capture signals from an early stage of olfactory processing in mice, and machine learning techniques to form a sensitive and selective chemical detector.
As a proof of concept, we have demonstrated that the system is capable of detection odors at concentrations comparable to that achieved by a trained animal. It can classify multiple odorants and concentrations. Current efforts are focused in developing the system towards detection and classification of real-world targets to investigate whether it can be used, for example, to find explosives or diagnose diseases.
Previous work
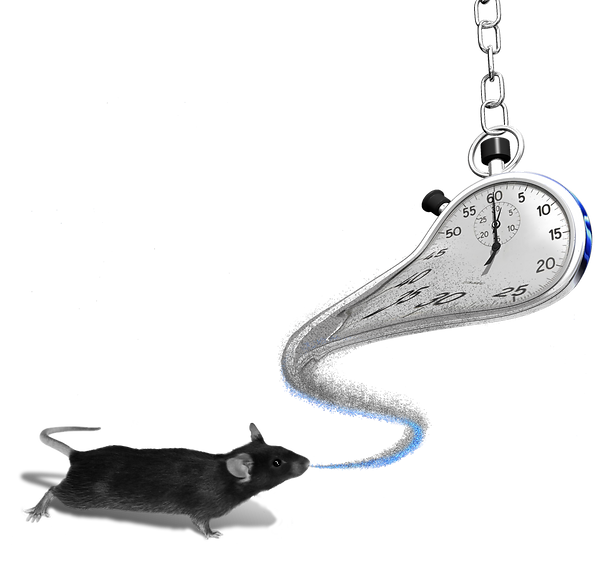
Timing in olfaction
Our lab's early, foundational work focused on unraveling the
temporal aspects of olfactory information processing using psychophysics, electrophysiology, and optogenetics.
Contrary to the common belief, we demonstrated that olfactory processing is temporally very precise at both
the levels of the neural code (Shusterman-2011) and
of behavior (Smear-2011, -2013), and also that it
can be very fast: mice can make olfactory decisions
in less than 100 ms (Resulaj-2015).
Odor identity coding
Humans can easily identify visual objects independent of their position and lighting, words in speech independently of their volume and pitch, and smells independently of odor concentration or the presence of background odors. Recently we propose a new model for concentration invariant odor coding, Primacy coding model (Wilson-2017). According to this model, the odor identity is encoded only by a small number of the most sensitive receptors for a given odor. Higher concentration of an odor excites larger number of receptors/glomeruli. So, the common feature across different concentrations is a set of glomeruli with highest affinity to a given odor, which are excited at all concentrations. We assume that those glomeruli are excited first during an odor inhalation (see figure). Thus, among all excited glomeruli for a given odor, the glomeruli excited first should have a preferentially higher relevance for odor recognition.
The strong evidence for this model has been recently obtained in series of experiments in the lab. Mice were train to discriminate between two odors independently of their concentrations. Using temporally precise optogenetic masking of OSNs, we established that a mouse can discriminate odor identity using only information presented during a small temporal window at the beginning of the sniff cycle. This window is much shorter than the time at which most glomeruli are activated. So, animals were able to make a concentration independent odor discrimination based on signals from very few first glomeruli (Wilson-2017)
Primacy coding model:
